Annotation.
This paper
describes a new approach to explaining the phenomenon of the charge cluster,
discovered by Kenneth
R. Shoulders (K.Sh.) at the end of the last century. The proposed
model is not based on any controversial scientific hypotheses, but is
consistent with generally accepted norms of science. The exception is the
assumption of the possibility of short-range forces for electrons, contrary to
the position of the "standard model". The concept explains the
physics of the observed LENR reactions, as well as interprets the nature of the
triboelectric effect from a new perspective.
New terms and abbreviations.
Electronic
crystal (El.cr .)
Charge
cluster (Ch.cl .)
A charge
cluster in the "hot" phase (hot-Ch.cl .)
A charge
cluster in the "cold" phase (cold-Ch.cl .)
The digital values used
-the number
of electrons in the cluster is 10^8...10^11 pieces (according to measurements
K.Sh .)
-the number
of atoms involved is 10^3... 10^6 pieces (according to measurements K.Sh .)
-cluster
diameter 10^-7 m
(measured by K. Sh.)
-diameter
of the electron crystal 1.18*10^-9
m (calculation)
--the
lattice pitch of the electronic crystal El.cr . 2.05*10^-13 m (calculation)
-the
diameter of the helium atom is 0.62*10^-10 m
-the
diameter of the nitrogen molecule is 3.2*10^-10 m
-the
average distance between air molecules under normal conditions is 10^-8 m
-the
diameter of the nucleus of a nitrogen atom is 3.1*10^-14 m
-the
diameter of the electron is 10^-18
m
-the mass
of an electron is 9.1*10^-31
kg
-protons
and neutrons are about 1800 times heavier than an electron
-the
electron charge is -1.6*10^-19 Kl
-the
wavelength of the blue color is 5*10^-7 m,
--the
ionization energy of the nitrogen molecule is 15.6 eV
Historical background.
Physicists, when working with arc
discharges in vacuum and gaseous media, have long paid attention to atypical
spark formations and small ulceration on the anode, always of the same
characteristic shape. Winston Bostick
met Kenneth R. Shoulders (K.Sh.) at a conference in San Francisco, Diego on November 10, 1980 and
interested him in this incomprehensible phenomenon. After that, K.Sh. worked
purposefully on this topic in his laboratory for many years and advanced in
this direction so much that he can deservedly be considered the discoverer of
the Charge cluster (Ch.cl.). The atmosphere of this work is conveyed in his
autobiographical essay "EV A Tale of Discovery" in 1987, and detailed
information on the research equipment he developed can be found in the
description of his patent US5123039 "Energy Conversion Using High Charge
Density" in 1992.
K.Sh. was able to isolate these
formations from the complex process of arc discharge in a gaseous medium, study
them with the help of his own equipment and evaluate their qualitative and
quantitative characteristics. In fact, he discovered a new phenomenon – the
ability of electrons to pass under certain conditions into a state of group
anomalous density, into a kind of condensed state. The main condition for the
formation of a bunch of such electrons is a high level of electric field
strength. In his laboratory, K.Sh. obtained such a field on the tip of a
needle-shaped cathode, additionally treated with a liquid conductive material.
In nature, conditions for the formation of such clusters can occur on the sharp
edges of mineral or ice crystals, as well as, possibly, in some biological
forms.
According to K.Sh. data, a single
Ch.cl., or as the author calls it EVO, has a size of about 0.1 microns, and the
number of electrons packed into such a cluster is 10^8...10^11 pieces. At the same time, the charge cluster
captures atoms of matter from the surrounding space in the form of positive
ions in the amount of one per 100,000 electrons, i.e. 10 ^ 3 ... 10 ^ 6 pieces.
Interestingly, this formation as a whole turns out to be almost electrically
neutral, despite such an imbalance between electrons and plus ions. The energy of Ch.cl. is also impressive - it
glows at the stage of formation and forms a crater when destroyed at the anode.
In the description of his patent US5123039 on page 68 (line 16), Kenneth R.
Shoulders (K.Sh.) explains the extraordinary energy intensity of the charge
cluster (Ch.cl.) in this way:
«The source of this increased energy
appears to be the vacuum zero point energy, or zero-point radiation. An EV, as
a coupling device to zero-point energy, operates as an energy conversion
mechanism whereby high frequency Zero point energy of the vacuum continuum is
converted to lower frequency energy, captured as electrical output energy by
the traveling wave conductor, for example.»
This interpretation of the nature of the
phenomenon explains little, it is counterproductive. Meanwhile, if we assume that
the experimental results correspond to reality and the condensed state of
electrons in nature takes place, then we can build a completely acceptable Chcl
model that will not contradict the generally accepted norms of science.
Critical position
All
further constructions, explanations and conclusions of the author are based on
the discovered K.Sh. phenomenon, on its EVO, which exhibit very contradictory
properties. Indeed, a certain luminous macroparticle observed with an optical
microscope includes 10-11 pieces of electrons and only 10^ 6 pieces of atoms
and behaves like an almost electrically neutral object. How is this possible,
how can the protons of these few atoms shield such a powerful negative charge
of electrons? To do this, they must be far enough away from the electrons, and
those, in turn, must go into a condensed state and be concentrated in a
microscopic volume in the center of the formation. To explain the phenomenon,
one of the statements of the physical "standard model" had to be questioned,
that electrons are a simple "point particle" and, as representatives
of the leptone class, do not have short-range forces.
An electronic crystal.
(El.cr.)
In his routine experiments, K.Sh.
obtained charge clusters by applying a relatively small pulsed negative voltage
to the cathode of the diode under vacuum conditions with a slight addition of
inert gas. At the same time, an electric field of very high intensity appeared
on the pointed electrode due to the insignificance of its area. This turned out
to be enough to start forming condensed clumps of electrons. At the same time
and along with them, a glow discharge and free electrons were recorded in the
experimental zone. This fact suggests that the newly formed electronic crystal does
not carry any significant amount of energy. He will begin to acquire it
immediately after its appearance, gradually turning into Ch.cl., and solely at
the expense of the surrounding space.
Let's look at how electrons condense into
compact, crystalline clumps - electronic crystals (El.cr.). The mechanism of
this process is explained by the figures given here, which need to be
considered together. Figure 1 shows the force field near the electron from the
position of a single test charge of negative polarity. The resulting force
action on this charge (the red curve) consists of the action of three forces:
Q, R and S (conditionally). The first is the usual Coulomb field force,
repelling our test charge with a force inversely proportional to the square of
the distance. The positive R and negative force S are short-range forces, they
decrease very quickly with distance. Their physics is not discussed here.
As we approach the electron, the effect
of the field on the test charge initially obeys the Q – law. Then a positive R
–field begins to act and the force repelling the test charge from the electron
first decreases, then reaches zero (point 3), and finally changes sign. Now the
test charge is attracted to the electron and it needs to be held. The force of
attraction increases to the value bb, after which it decreases again. A
powerful negative field S begins to act, it is the closest acting one. At point
2, all forces balance each other, and the charge trapped here is in a potential
well. To get out of it, you need to expend energy.
|
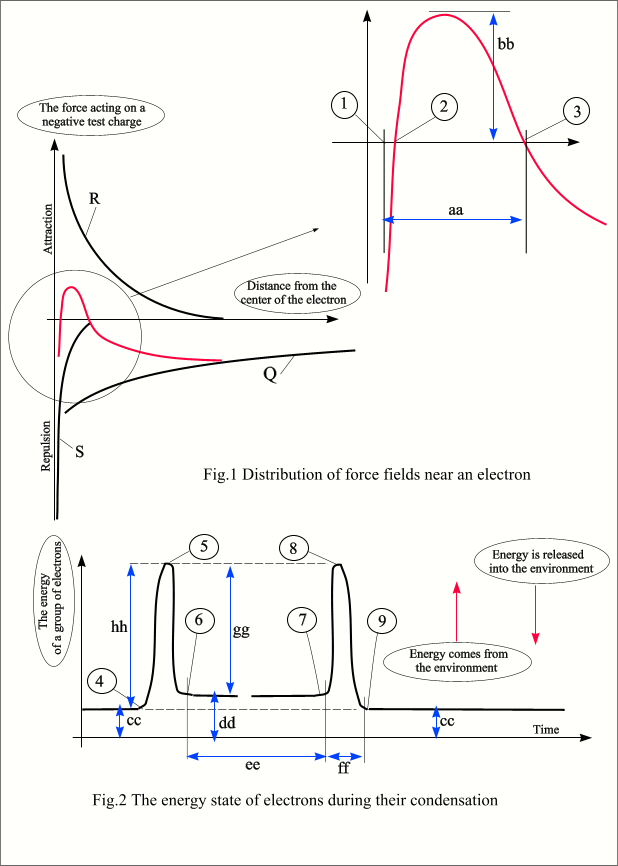
|
Everything that is said about the test
charge also applies to an electron approaching another electron or a group of
already condensed electrons. Having spent the work on bringing the electron
closer to such a group, we will immediately partially return it when the
electron "gladly" joins the group under the influence of a powerful
R–force. The electrons in the condensed group, as in a solid crystal, are
pulled together by a powerful field. According to the interpretation in Fig.1,
they are located at point 2 and can fluctuate to the right and left relative to
this point in their thermal motion. The convergence of electrons is
counteracted by a very sharply increasing S –force, the crystal is practically
incompressible (point 1). But it is able to stretch significantly (point 3),
showing the property of a certain heat capacity. This will be useful when the
condensed electron crystal, already mature for LENR manifestations of hot–Ch.cl.,
will have to store and disperse significant energy.
Figure 2 shows the change in the energy
state of the system during the formation and then destruction of a condensed
group of electrons. Suppose, at the tip of the cathode of a vacuum diode, with
the help of a concentrated electric field, the electrons in a sequential
process are supplied with the energy hh necessary for their rapprochement with
the group. Immediately, a significant part of the gg energy returns to the
reaction zone. As a result, the condensed electron crystal has a relatively
small internal energy dd compared to the initial cc. There is no need to
confuse the energy of this crystal with the energy of the hot-Ch.cl. formed
from it. The charge cluster collects its main considerable energy from the
ionized environment, as it will be described later. The ee segment is the
lifetime of the electronic crystal. A deep potential gg pit can preserve it
indefinitely under certain conditions. In our case, under the conditions of an
experiment with a vacuum diode, a condensed group of electrons at point 7
approaches the anode and ceases to exist. The reverse, also sequential process
occurs: the anode transfers energy gg to each electron and immediately takes
energy hh from it.
In nature, condensed electronic crystals
cannot exist in their pure form; with the participation of positive ions, they
first turn into hot-Ch.cl., and then degrade into cold-Ch.cl..
Formation of a charge cluster (hot-Ch.cl .)
A clot (El.cr.) of one hundred billion
electrons (10^11) creates a powerful local electrostatic field that attracts
positive gas ions from the close environment. Accelerating in this field, such
an ion can acquire energy in the millions of electron volts (MeV), while the
energy of ionization, or separation of an electron from an atom from its upper
orbit, lies in the range of 10-20 electron volts. Our atom crashes into a dense
bunch of electrons and passes through it. At the same time, it loses all its
electrons and, in the form of a naked atomic nucleus, as in a hot plasma, now
with a charge equal to the number of its protons, continues to move in a
retarding electrostatic field. Due to the remaining energy, the nucleus flies
away some distance from the cluster and then begins to make elastic harmonic
oscillations without loss of energy through the focus of our electron cluster.
This means that, averaged over time, the geometric center of the initial
electric charge is now in the focus of the resulting hot-Ch.cl., that is, in
fact, a single positive charge has moved from the distant periphery towards a
powerful formation charged with the opposite sign. The perfect work is
transferred to the kinetic energy of the oscillating nucleus, and this energy
already belongs to the macro object hot-Ch.cl..
In our case, a cluster can attract one
million such positive ions, the process continues until a dynamic shielding
layer of positively charged atomic nuclei appears around the electron cluster,
spending most of the time in the peripheral zone of the cluster. If the
electrons remaining from the ions that flew through the El.cr. are not included
in the crystal, then they are carried away by an electric field to the sides,
simultaneously deionizing positive gas ions in the near environment. These ions
would not be able to pass through the El.cr. due to their low energy, and
having become neutral molecules, they no longer participate in the process. The
mutual repulsion of ion nuclei inhibited at the periphery will ensure their
strictly uniform distribution over the spherical surface of Ch.cl.. This, in
turn, will create an ideal symmetry of the entire cell and precise alignment of
the position in the focus space, through which atomic nuclei fly at high speed
from different directions.
Since, according to Coulomb's law, the
dependence of the force of action on the test charge on the distance is
quadratic, a relatively small number of positive ion nuclei can create the
illusion of its electrical neutrality near the surface of the cluster, i.e.
shield the El.cr.. If we imagine our object as a model in which the shielding
layer is replaced by a hollow sphere with a diameter of D, and an electronic crystal with a sphere
with a diameter of d, then this diameter can be calculated as follows.
It is also easy to calculate the average
density hot-Ch.cl. - it will be at the level of popcorn or cork.
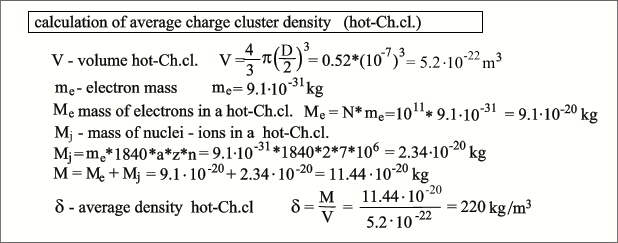
As well as the step of the El.cr. lattice,
as can be seen, the core of even a heavy element can easily fly through such a
lattice, but it is completely impassable for an ion.
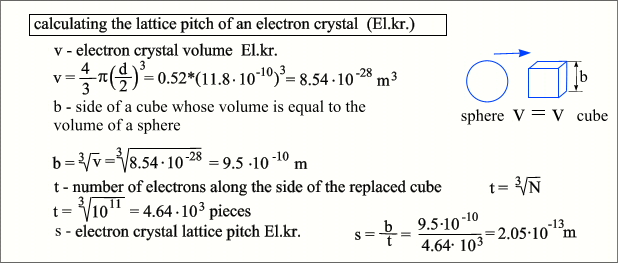
If you
imagine the sizes of objects on a logarithmic scale, you can clearly imagine
the size relationships and conditions for the existence of hot-Ch.cl. from the
following figure.
Now let's calculate what
energy the ion of the nitrogen molecule N2+ (q) will receive, accelerating in
the field of a small El.cr. consisting of 10 ^ 9 electrons (Q). According to
Coulomb's law, the force acting on charges is inversely proportional to the
square of the distance: |
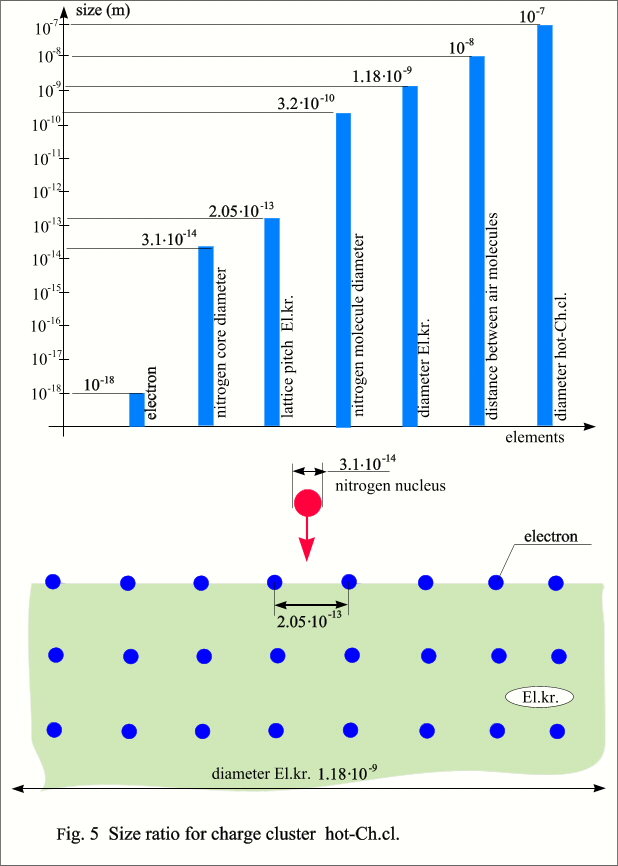
|
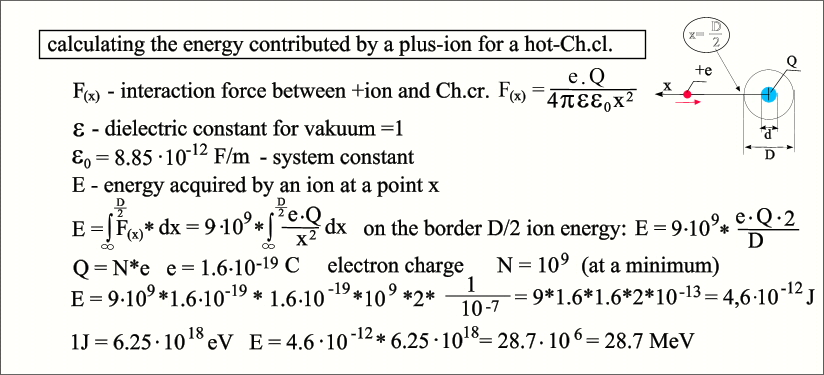
The ion
received energy of 28.7 MeV at the hot-Ch.cl. boundary, at the coordinate x =
5*10^-8. Meanwhile, the ion continues to accelerate until it comes into contact
with a crystal – a bunch of electrons whose diameter is significantly smaller.
For example, if it has a diameter of 10^-8 m, the energy and it will increase by an
order of magnitude. How large is the
kinetic energy of a charged particle accelerated by a field and what will its
interaction with a bunch (crystal) of electrons lead to?
I will indicate in the same electron
volts the energy intensity of the processes occurring in the operating area of
the electric arc process, as well as in more serious phenomena:
- the
energy of motion of a gas molecule (average) at a temperature of 1000 degrees K
0.13 eV
- the
radiation energy of the visible light quantum is 2 – 3 eV
- the
energy of loss or acquisition by a valence electron molecule is 5 – 20 eV
- the
energy of total ionization (up to the bare core – ion) of the helium atom is
78.98 eV
- the same
lithium atom 203 eV
- the same
beryllium atom 402 eV
-
the energy
of convergence of two protons to overcome the Coulomb barrier 1.1 MeV
- the same
for nitrogen nuclei of 70.6 MeV
- the same
for 700 MeV uranium nuclei
For the sake of persuasiveness, we
calculate the Coulomb barrier for nitrogen using the same formula:
Ek = (9⋅10^9 * 7 * 7 *
(1,6⋅10^-19)^2 ) / 10^-15 = (9 * 49 * 2.56)⋅10^-14 =1.13⋅10^- 11 J
Ek = 1.13⋅10^-11 / 1,6⋅10^-19 = 7.06⋅10^7 eV = 70.6
MeV
When experimenting with a gas vacuum
diode, there are probably many small El.cr.’s, what happens to them? When
1840*14 electrons weigh the same as one nitrogen ion, and the electrostatic
field directs these particles strictly into each other's forehead, the crystal
cannot withstand, it crumbles into electrons. And
only if the mass of the El.cr. is many orders of magnitude greater than
the mass of the one crashing into it, does the process become possible when the
N2+ ion accelerated to high energy will leave its shell at the boundary of the
electron crystal, fly through the center of the electron cluster and turn into
a core ion with a charge of 7+. A sufficient number of such ions will turn such
a cluster into a kind of "reverse atom" formation, in which not
electrons move in circular orbits around the nucleus, but nuclei make harmonic
oscillations through the focus of the electron crystal in the center. This
model allows us to explain the mechanics and energy supply of nuclear LENR
reactions.
Nuclear LENR reactions inside the hot-Ch.cl..
The main advantage of hot-Ch.cl.’s is
their ability to implement cold fusion reactions (LENR processes). The main
argument of the opponents of cold nuclear fusion is the impossibility of
overcoming the Coulomb barrier at low temperatures. However, at these low
temperatures, physicists on simple laboratory accelerators are constantly
experimenting with nuclear reactions, accelerating a proton to energies above 1
MeV and directing it at a target. Created by nature, hot-Ch.cl. provides a
similar, but purely natural mechanism for the implementation of cold
thermonuclear reactions, the effectiveness of which consists of two principles:
- high-energy nuclei and precise alignment. All atoms involved in hot-Ch. Cl.
exist in the form of "naked" nuclei – ions, their electrons are
transferred to a condensed electron clot. The nuclei continuously make harmonic
oscillations through the local hot-Ch.cl. focus, while at the cluster focus
their energy completely transforms into kinetic energy and they pass this point
at a very high speed.
The probability of close interactions of
nuclei moving from different directions and not synchronized in time is quite
high. At the same time, since we are talking about naked nuclei, there is an
exceptionally elastic exchange of impulses, as a result of which the nuclei
change speeds and directions. Naturally, there is a certain alignment in the
distribution of energies, and there is a probability of an event when two
energetic nuclei on opposite courses collide in the very focus of hot-Ch.cl..
This will no longer be an elastic collision, the Coulomb barrier has been
overcome, the nuclei will merge with the release of energy or split in a
different ratio with the absorption of energy, a nuclear reaction will occur.
Now about the accuracy of guidance;
let's assume that hot-Ch.cl. is built according to the laws of spherical
symmetry. The core ion, coming to the surface of hot-Ch.cl., completely loses
the radial component of its kinetic velocity. If there is a tangential
component, as a result of random interaction during the last flight of the focus,
then it is removed by electrostatic repulsion of other nuclei – ions currently
located on the same spherical surface.
Therefore, the core stops completely in space before the next movement
to the center of hot-Ch.cl, and its trajectory is not distorted in any way and
is always directed strictly into focus - the electric and geometric center of
hot-Ch.cl.
When a nuclear reaction occurs, a
powerful case of 10^11 electrons gently dampens possible fast particles and
hard radiation, converting their energy into heat. At the same time, the focal
center is temporarily blurred, making the probability of a new meeting of the
nuclei insignificant for a while. The
nuclear reaction of two medium-sized nuclei cannot produce such energy as the
fusion of deuterium and tritium, but some small mass defect is released, and
this energy supports the current radiation needs of the charge cluster.
Reactions can often occur with a zero or negative energy balance. In the
process of vital activity, hot-Ch.cl. continuously exchanges matter with the
environment - new atoms and molecules are involved in the charge cluster,
others are released into the environment and are essentially a product of
transmutation. Under favorable
conditions, a moving hot-Ch.cl., due to nuclear reactions with a preferably
positive energy balance, can do a lot of work: the formation of known craters
in metal foil during its destruction, making moves in photoemulsion and even
denser materials (strange radiation).
Degradation of the charge cluster,
transition
hot-Ch.cl ...>>… cold-Ch.cl.
In the drawing, you can see two forms of
existence of the same object, and they do not contradict each other. It is
based on the El.cr. electronic crystal, a small, spherical object linked by
short-range forces with a huge negative electrostatic charge. Not being in an
absolute vacuum, this charge will be compensated in one way or another, a
protective shielding shell will appear. The most interesting is the hot form
hot-Ch.cl . This form allows for the possibility and explains the mechanism of
the LENR process in the form of a variety of nuclear reactions. Not very rare
and unlikely thermonuclear reactions of hydrogen and helium, which are realized
in the hot plasma of the sun, but full-fledged head-on collisions of any nuclei
involved in the process. The
"cold" form of cold-Ch.cl. differs in that the shielding is carried
out by slow thermal ions with a charge plus one e.
|
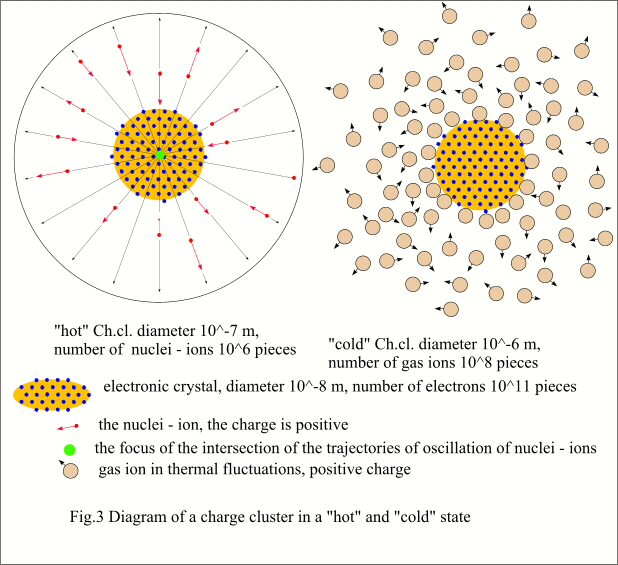
|
This form can occur immediately upon the
birth of an electronic crystal if the pressure in the chamber is high enough
that the free path of the molecules does not allow the ions to accelerate
strongly in the electrostatic field of the crystal. The formation of such a
cluster is also possible through the degradation of its "hot" form.
The phenomenon of finding an array of electrons in the immediate vicinity of a
cluster of positive ions is due to a potential barrier of about one hundred
volts, preventing the transition of an electron from the lattice of a crystal
to the valence level of an atom. (the energy of a single ionization of an atom
is only 5 – 20 eV). The figure "100" follows from K. Sh.'s
experiments to reduce the voltage required for generation hot-Ch.cl . The
diameter of the "cold" cluster is significantly larger, on the order
of 10^-6 m,
and the number of attracted ions is 10^ 7 - 10 ^8, respectively. This is due to
the fact that most of the shielding component is not as far from the electron core
as in its hot form.
Let's take a closer look at the formation
process cold-Ch.cl. under conditions of relatively high gas pressure in the
experimental chamber. The electric field at the cathode forms an electronic
crystal. According to Fig.2, the energy hh is transferred to the next electron,
for example, 100 eV. At the same time, it is pressed against the electronic
crystal, short-range forces are turned on, the energy gg, say 95 eV, is
returned to the cathode and this electron is included in the crystal lattice.
The dd – cc difference of 5 eV per electron is the acquired positive potential
energy of this new group object in relation to the environment. 95 eV,
respectively, is the energy that needs to be expended in order to detach the
extreme electron from the crystal. At some point, the crystal separates from
the cathode, acquires a symmetrical shape and rushes to the anode, following
the electrostatic field.
In a discharged gas environment, there
are always positively charged ions, they "squeeze" to the electronic
crystal, surround it and, unable to tear off the valence electron, gradually
shield the charge of the crystal. If the electric pulse at the cathode was
short, or the experimenter artificially created a highly ionized plasma in the
chamber, the Ch.cl. it will not have time to discharge at the anode. Actually,
there may not be an anode in the chamber at all, the circuit can work on a
capacitive load, only a collector is required to collect free electrons.
How and how long can he live hot-Ch.cl.
what is its degradation? If the cluster is large enough and nuclear reactions
occur in it, then there are no problems with replenishing the average kinetic
energy of oscillating nuclei. Newly formed
nuclei and nucleons with high velocities as a result of the reaction share
their kinetic energy as a result of elastic collisions with other nuclei. . The
fastest cores are leaving space Ch.Cl. In doing so, they ionize neutral atoms,
filling their electron shells.
New positive ions of atoms arrive in
place of the nuclei that have left the cluster. There is an intensive exchange
of material between hot-Ch.Cl. and the environment. The excess electrons are
displaced by the field of the electron crystal to the periphery, where they
reunite with positive gas ions, and additional energy is withdrawn from the
reaction zone. Life time hot-Ch.Cl. It depends, first of all, on the
composition of the environment in which it exists, on those nuclei that form
its body.
Under favorable conditions, the
proportion of nuclear reactions with a positive energy balance prevails, and
the cluster exists for a long time. The density of the gas that surrounds the
cluster also plays an important role. Positively charged ions do not cause
difficulties for nuclei flying to the periphery. Neutral atoms, despite the low
probability of collision with them (the diameter of the core - ion is 10^-15 m, the diameter of the atom
is 10^-10 m,
the average distance between air molecules under normal conditions is 10^-8 m), lead to hot-Ch.Cl. energy
losses and, eventually, to its extinction, the transition to the stage
cold-Ch.cl..
LENR manifestations are possible only in
large Ch.cl. In which the average energy of the ion nuclei approaches 0.1 MeV,
they can be obtained in the laboratory, in thunderstorm or dust clouds, or
under special conditions. Most often, Ch.cl.’s are small in size and do not
carry much energy, they do not glow and are difficult to register. Hot-Ch.cl.
It has a diameter of 10^-7 m,
and naked positively charged atomic nuclei (size 10^-15 m) spend most of their time
accelerating and decelerating in their vibrations on its surface and the upper
third of the volume. The air molecules have a size of 10^-10 m and are 10^-8 m apart at normal pressure.
The
scale ratios of the design under consideration suggest that hot-Ch.cl. does not
have a serious aggressive impact on the environment. In order to destroy the
orbital shell of a neutral atom and capture its electrons, a positively charged
ion atom must approach this atom at a distance less than the diameter of the
atom. As can be seen from the model, the probability of such an event is low,
and therefore neutral air molecules can freely move in their thermal motion
through the Ch.cl. structure, this cannot be said about free electrons - the
nearest ion atom will capture a wandering electron and immediately deliver it
to a clot of condensed electrons.
Some usage examples Ch.cl.
Hot-Ch.cl.
is more about LENR processes, about getting cheap energy, but the prospects for
using cold-Ch.cl. are better viewed. Although no one denies the results of the
experiments of Winston Bostik and Kenneth R. Shoulders, no one is in a hurry to
repeat and develop these experiments, prove the existence and investigate the properties
of these very Ch.cls. But since this is only a hypothesis, let's fantasize a
little and mentally make three products from this cluster, and then see how
these products can be applied for the benefit of society.
Product "A". Cold-Ch.cl is an
electrically neutral macro object consisting of gas ions, which are
additionally compacted by the forces of attraction to an electronic crystal
located in the center. At atmospheric pressure, such clusters will be heavier
than air, although they consist of the same nitrogen and oxygen. We will
concentrate them and collect them in the form of a liquid at high pressure and
low temperature.
Product "B". Here we are talking
about the same condensate, but a heavy inert gas or mercury vapor is chosen as
the shielding ions of the electronic crystal. In this case, apparently, we will
get a liquid or pasty preparation at normal pressure and temperature.
Product "C" is more difficult to
obtain. Here it is necessary to fix cold-Ch.cl. on a silicon substrate,
carefully neutralize the positive gas ions with a soft flow of electrons. In
this case, the gas molecules will fly away, and the size of the clusters will
decrease by two orders of magnitude. Pure electronic crystals will be fixed on
the substrate by an external electric field. Further, by vacuum spraying of the
dielectric, the crystals are integrated into the substrate. We get a plate with
a constant surface charge or even volume in coulombs per unit area. What will
the use of such new materials do for the development of technology?
The most obvious use of composites from
electronic crystals. Like a neodymium magnet, strips with a powerful negative
electric charge are arranged along the generator on the rotor of an electric
motor, a positive potential is applied to the flat electrodes of the stator in
the desired sequence. Such an engine is much lighter, it does not use
transformer steel necessary for electromagnetic induction, copper is also
consumed at a minimum, since there are no strong currents and no windings
either.
Now about
energy storage in industrial super capacitors and using other devices. In a
capacitor, energy accumulates not in the form of complexes of chemical
compounds formed during battery charging, but directly in the form of an
electric charge, that is, in the form of living electrons artificially spaced
on different capacitor plates. The more of these electrons (linear dependence)
and the greater the potential difference we spread them (quadratic dependence),
the more energy there is in the capacitor. Consider an ordinary capacitor fig.4
– "a". When charging it, an external energy source moves electrons
from one capacitor plate to another, overcoming an increasing potential
difference.
|
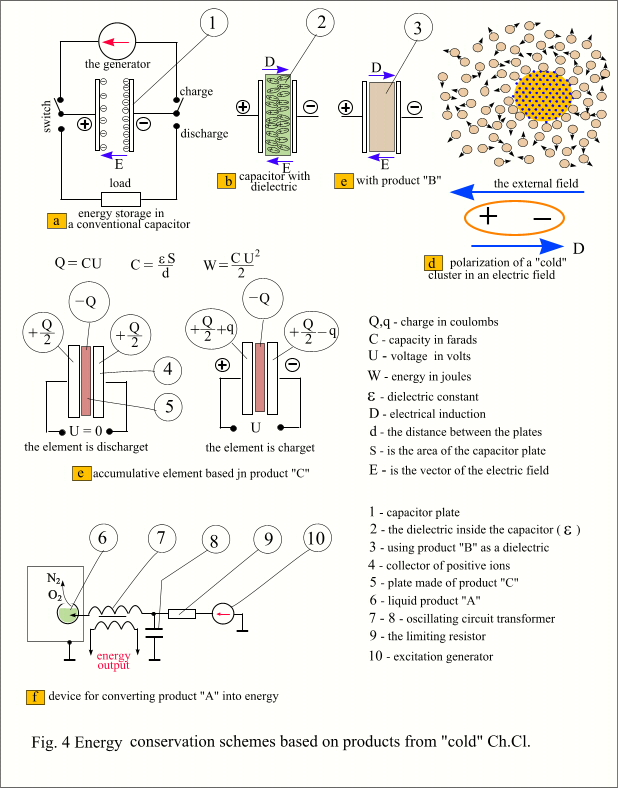
|
The
capacitor is characterized by the "strange" behavior of electrons.
Under the influence of external influence, there is no uniform compaction of
electrons in the entire volume of the conduction band of the metal plate of the
capacitor, and they focus exclusively on the surfaces directed towards each
other. The electric field does not penetrate into the conductor, and the
capacitor lining can be made in the form of a metal film of micron thickness.
The only possible way to increase the capacitance of a capacitor is the dielectric
constant of the material through which the lines of force of its electric field
pass. Otherwise, the capacitor remains hostage to its geometry. (formula 2 in the figure). If energy is
stored in a vacuum capacitor as a result of forced compaction of similarly
charged electrons in a certain closed geometric space, the dielectric itself
additionally stores energy in a dielectric capacitor. (see Figure 4b). The
elastic internal forces of the material under the action of the field allow
asymmetrically polarized molecules to shift and unfold, partially compensating
for the external field. During the discharge of the capacitor, this energy is
returned. The gain is 8 times for porcelain, 10 times for aluminum oxide in an
electrolytic capacitor and 24 times for alcohol. (relative permittivity ε ). There are specific materials,
for example, ferroelectrics, with an ε equal to tens and hundreds of
thousands of units. (feel the difference). However, their use for the purpose
of energy conservation is difficult due to strong hysteresis and residual
phenomena in the material.
In Fig. 4c Our product "B" is
used as a dielectric. It is enclosed in an insulating shell to exclude the
contact of weakly bound ions directly with the capacitor plates.
"Cold" clusters based on a heavy inert gas under the action of an
electric field form a homogeneous mixture of free-floating electronic crystals
in a liquid of massive ions. Such a structure, due to its plasticity, is
apparently capable of accumulating significant energy.
The storage element based on product
"C" is shown in Fig. 4e. This is not a capacitor, but a system of
charges opposite in sign and always equal in magnitude, located in space
opposite each other. In the middle there is a silicon wafer, for example, with
pure electronic crystals embedded in it with the highest possible density
(product "C"). This is an insulator, the movement of current carriers
through this material is impossible. Positive ion collectors are located on
both sides of the plate (Fig. 4e position 4). The properties of these
collectors are such that, under the action of the electric field of the central
plate, they will be filled with positive ions, the total charge of which will
be equal to the central charge. Each collector has an electrode through which
the external electrical circuit of the element is closed. When the charges are
balanced, the potential difference at the terminals of the element is zero.
The element is symmetrical and can be
charged in any direction. When voltage is applied from an external source,
electrons discharge positive ions at the electrode of one collector and create
new positive ions at the electrode of another collector (if it is a gas
collector, then they simply discharge the existing negative ions). The ion concentrations to the right and left
of the central charge change, a compensating electric field appears between the
collectors and, accordingly, a potential difference occurs at the terminals of
the element. A device based on a certain volume of artificially ionized gas, an
electrolytic bath or a solid semiconductor with hole conductivity can be used
as a collector of positive ions.
Now about using product
"A". In fact, it is just air,
it is ionized, and the resulting electrons are collected into electronic
crystals, around which the same charged ions are grouped. The energy stored in
this product consists of the energy of
dd-cc (Fig. 2,) and the ionization energy of air molecules (based on
each electron of the crystal). The potential barrier gg is responsible for the
stability of the cluster, which must be overcome in order for the hh energy to
be released and the released electron to reunite with its ion. For such a
waste-free "burning" of air in the air, a special device will be
required (Fig. 4f).
When Chcl is discharged in a vacuum chamber on a strongly positive anode, the
instantly released energy creates a microcrater of molten metal. In the device
shown in the figure, the oscillating circuit allows you to discharge the
crystal in portions and divert the received energy to the consumer.
Adhesion, triboelectric effect.
Adhesion
(from Latin adhaesio – adhesion, adhesion, attraction),- this is the connection
between heterogeneous condensed bodies in their molecular contact.
It becomes
obvious that the ubiquitous cold-Ch.cl.’s in nature have a decisive influence on this
phenomenon. As for the triboelectric
effect, there is still no clear explanation for this phenomenon and charge
clusters put a lot in their place.
All hot-Ch.cl.’s after the active phase of their existence
gradually move into the stable stage of the "cold cluster". At the same time, we have the following
picture: a very small electronic crystal of ~ 10^-9 m (about ten atomic
diameters) is surrounded by a huge cloud of single-charged gas ions. The deionization
potential of such an ion is not enough to detach an electron from the surface
of an electronic crystal and thereby begin its disassembly. A powerful
electrostatic field tightly presses the nearby ions to the crystal. Further,
the ions are arranged more loosely, and thermal motion becomes active. The
attracted ions turn out to be orders of magnitude more than in the previous
phase of the "hot" cluster. That is, all the conditions for the
long-term existence of this, in fact, lump of air appear.In general,
the small internal potential energy of these formations remains unclaimed, it
cannot be released under normal conditions.
Let's see how the interactions develop
between a powerful point negative charge of an electronic crystal, a cloud of
single-charged air ions and a solid or liquid surface to which Ch.Cl has a
tendency. In electrostatics, the effect of the field is inversely proportional
to the square of the distance, therefore, the further the ion shell that
shields the point charge extends, the less charge it can have. Shielding refers
to the compensation of the electric field of our cluster in a relatively close
environment. With more distance, the object will still be perceived as negative
and will attract positive ions. Thus, the ion density will decrease with the
radius, and at the periphery the ion bond will be very weak. The property of a
"cold" cluster to adhere to any solid or liquid surface, regardless
of how electropositive or electronegative it will be, can be explained as follows.
When the cold-Ch.cl. hits an
electropositive surface, for example, a quartz grain, the electronic crystal of
the cluster shifts slightly towards this surface, resulting in an electrostatic
force of attraction. That is, Ch.cl. is capable of polarization. At the same
time, on the opposite side of the cluster, the field weakens and some of the
positive ions leave the cluster zone. Now, if the cluster is torn away from the
surface by some force, for example, as a result of friction, the number of
positive ions in it will be less and it will be negatively charged as a whole. This
is the main mechanism of the triboelectric effect. For an electronegative
surface, we have a mirror process - the electron crystal moves away from the
surface, the cluster attracts additional ions from the surrounding space, and
when the particle is detached, it acquires a positive charge. When we rub a
glass stick with a silk handkerchief, depleted and enriched with positive ions
charge clusters mix, and each side receives a part of the "foreign"
particles.When
charged bodies are separated, a potential difference arises, which is perceived
as triboelectricity.
Static electricity, electrification by
friction, thunderstorm manifestations are so common and widespread phenomena that
the explanation of their nature with the help of Ch.cl. is alarming. Then we
must admit that cold-Ch.cl. clusters are ubiquitous and we are so used to them
that we simply do not notice them. Then we must admit that in the nature around
us there is a certain excess of electrons over nucleons, because each Ch.cl.
contains an E.cr. of electrons, the number of which is orders of magnitude
greater than ions. It can be assumed that there is a very small excess of
electrons on the surface of our planet compared to the number of protons. The
reason for this may be the solar wind. A stream of corpuscles and ions coming
from the sun enters the Earth's atmosphere; on the other hand, even more ions
and neutral atoms are continuously blown away by the same solar wind from the
periphery of the Earth's atmosphere. A certain balance is being formed in terms
of the total electrostatic charge of the planet. How will excess electrons
behave on the surface of our relatively cold planet if they encounter in their
path, in the vast majority of cases, self-sufficient, electrically neutral
atoms, molecules and compounds.
|